CANCER STEM CELLS: Redefining the Paradigm of Cancer Treatment Strategies
Abstract
Cancer has been known to arise from long-lived cells in the body and to possess properties in common with undifferentiated, embryonic cells. Recent findings of a population of cells in solid tumors resembling stem cells supports a stem cell model of cancer. A scheme in which all cancers initiate from “activated” stem cells helps bring together data from genetic, cell biology, and epidemiology studies. Cancer can arise from embryonic cells in the case of childhood tumors; hormone-activated stem cells in the case of breast cancer; and following chronic activation of stem cells caused by tissue damage. This scheme helps explain the failure of many cancer therapies, points out deficiencies in certain research approaches, and focuses the problem on a subset of cells that can be explicitly targeted, leading to more efficient therapy.
The Cancer Stem Cell Concept
The idea that cancer cells have properties in common with embryonic cells is an old one. Cancer cells divide rapidly and are immortal, as are embryonic stem cells. Embryonal carcinomas and teratocarcinomas have long fascinated cancer biologists as they are derived from embryonic cells and give rise to cells of many lineages. Beatrice Mintz showed in 1975 that teratocarcinomas could give rise to normal chimeric mice (1). Hamburger and Salmon demonstrated that a subpopulation of cells in a tumor will grow in soft agar, and termed these cells “tumor stem cells” (2). In 1993, Al Knudson proposed that the ability of many tumor suppressor genes to give rise to specific cancers could be explained by their effects on tissue stem cells (3). John Dick proved in 1994 that cancerous stem cells were present in acute myelogenous leukemia by isolating such cells and documenting their self-renewing capacity—the critical property of all stem cells (4). Additionally, alteration in the growth regulation of stem cells has been invoked to explain the role of the Patched (PTCH) gene in causing basal cell carcinomas in patients with nevoid basal cell carcinoma syndrome (5).
Thus, the term cancer stem cell has arisen to describe cells that represent a minor population of a tumor and have the property of self-renewal. Self-renewal involves the replication of a stem cell generating, in the simplest example, another stem cell and a cell committed to differentiate. After many cell divisions, a tumor arising from a single stem cell is composed of cancer stem cells and non-stem cells that have a limited lifespan. Cancer stem cells could arise from normal stem cells, from committed cells that acquire the ability self-renewal capability, or by the fusion of normal stem cells with cancer cells.
It has been the identification of a small population of cancer stem cells in solid tumors such as breast and brain, however, that has captivated the field (6, 7). These stem cells appear to be the only cells in the tumor capable of immortal growth and transplantation of the tumor. Perhaps more astonishing is the discovery in long established cell lines, even HeLa cells, of a minor population of cells with stem cell properties (8, 9). Many researchers now suspect all cancers are composed of a mixture of renewing stem cells and “committed” cells that continue to proliferate but have a limited life span. The implications of this concept are important for basic science and well as cancer therapy. The regrowth of many cancers following therapy could represent the survival of cancer stem cells, just as the patient’s hair follicles and hematopoietic cells recover, because of the survival of hematopoietic and skin stem cells. A fraction of cells in a tumor are known to survive radiation treatment and cytotoxic drug exposure (10). Stem cells express drug transporters, DNA repair systems, and are refractory to programmed cell death, all properties that would serve to allow a cancer cell to resist our efforts to eliminate it (11–13).
Normal and Abnormal Stem Cells
The earliest cells of an embryo are totipotent stem cells, able to give rise to all the cell types of the body. These cells differentiate into more restricted, multipotent stem cells and then to the further restricted tissue stem cells. All stem cells have the property of dividing and giving rise to additional stem cells as well as to cells committed to differentiation that eventually stop dividing or undergo apoptosis (14). Some stem cells, such as hematopoietic stem cells, continue relatively rapid division, to maintain the massive number of lymphocytes and red blood cells needed by the body. Other stem cells, such as those in the skin and colon, undergo a slow but constant growth, replenishing the tissue, whereas other tissue stem cells can remain quiescent to become activated when stimulated by tissue damage or hormone exposure. It becomes obvious then that the proliferation of all the different types of stem cells in the adult requires exquisite control and that aberrant regulation could be hazardous. Cancer stem cells can arise from two potential pathways: 1) a stem cell losing growth regulation could directly become a cancer stem cell, or 2) a mature (i.e., differentiated or committed cell) could acquire the properties of self renewal and become a cancer stem cell (Figure 1⇓). There is good evidence for both mechanisms, as well as the possibility that these two pathways might be utilized in differing proportions at different times within the tumor. In fact, transfection studies in which human oncogenes transform murine fibroblasts demonstrate that non-stem cells can be converted into cancer (stem) cells. An even more outlandish idea is that normal hematopoietic stem cells may fuse with tissue cells to generate cancer stem cells (i.e., the formation of an in vivo hybridoma) (15).
Two stages involved in cancer stem cell activation. Cancer requires the generation of an actively dividing or “activated” stem cell. This cell may be an actively dividing stem cell, a stem cell stimulated to divide by tissue damage or inflammation, or from an a differentiated (mature) cell acquiring the property of self-renewal. Mutations inactivating tumor suppressor genes (negative regulators of growth) or activating oncogenes (positive regulators) convert the activated stem cell into a cancer stem cell. Further mutations are required for tumor progression and metastasis.
Stem Cell Activation and Cancer
Activation of a stem cell can happen in three ways. 1) The stem cell may be naturally dividing, as in the embryo or the hematopoietic system; 2) the stem cell can be activated by hormone such as estrogen for breast and ovarian cells; 3) the stem cell may be stimulated to divide through tissue damage caused by inflammation, chemical exposure or infection. With regard to cancer stem cells, it becomes of central importance for researchers to understand how stem cells take this first activation step. A model of stem cell activation and cancer should take into account the many diverse types of cancer and their varied causes. Such a model in which stem cell “activation” is the key first step satisfies many observations (Figure 1⇑). Activation could occur through hormonal stimulation; tissue damage caused by inflammation, radiation, chemicals, or infections; or inactivation of certain tumor suppressor genes (Table 1⇓). An activated stem cell would be a target for further genetic events leading to autonomous growth, loss of cell cycle regulation, and resistance to apoptosis—all well understood properties of cancer cells (16). This model accounts for experimental observations on tumor initiators and promoters (17). Most of the environmental agents causing stem-cell activation would be classified as promoters, non-DNA-reactive agents that enhance the tumorigenicity of initiators (i.e., mutagens). Some environmental agents, such as tobacco smoke and UV irradiation, contribute to both initiation and promotion by stimulating tissue proliferation and damaging DNA.
Routes to Stem Cell Activation
Cancers have been proposed to derive from one of three distinct tissue types: embryonic, conditional growth, or renewal (3) (Figure 2⇓). Embryonic cancers arise from rapidly dividing embryonic stem cells; retinoblastoma is the prototype for this type of cancer (18). Retinoblastoma arises in retinoblasts, embryonic cells in the eye that are highly proliferative. The target cell is a naturally activated stem cell. The inactivation of the RB1 gene turns this embryonic stem cell into a cancer stem cell that ignores the growth regulatory signals that normally arrest the stem cell once the developing eye is filled with retinoid cells. Other childhood cancers such as Wilm’s tumor and leukemias arise from genetic events in kidney and hematopoietic stem cells, respectively. These types of cancer require the fewest genetic events, as the target cell is a fully activated stem cell. Stem cells are also activated by hormones and/or growth factors in conditional growth tissues, as described by Knudson (3). An example is the breast, which undergoes dramatic growth during puberty and is then stimulated by estrogen and other hormones monthly during the woman’s reproductive life (19). Activated stem cells in the breast would be the target cell for breast cancer. Inactivation of P53 and other genes would transform breast tissue stem cells into hyperplastic lesions, the target for further events leading to the progression of pre-malignant cells to malignancy (Figure 2⇓).
Carcinomas arising from activated cancer stem cells in different types of tissue. Embryonic tumors arise from normal embryonic stem cells. They are already rapidly dividing (activated) and require few alterations to become malignant. Conditional growth tissues such as the breast are activated by hormones during puberty and during reproductive life. Activated stem cells in these tissues suffering tumor suppressor gene mutations give rise to premalignant lesions progressing to malignancy. Renewal tissues such as the colon and skin have dormant or slowly replicating stem cells. Certain tumor suppressor gene mutations give rise to activated stem cells with uncontrolled replication. Subsequent mutations give rise to tumor progression.
Breast cancer is one of the best understood tumors; epidemiological risk factors, preventive and therapeutic strategies, and genetic data regarding this disease can be combined into a single scheme (Figure 3⇓). The major breast cancer risk factors involve hormonal and reproductive events (20). Early onset of menarche increases cancer risk, whereas pregnancies decrease risk. Each of these factors influences either the number or activation of breast stem cells. The major prevention strategies currently available similarly affect either estrogen production or its action on cells. Inhibition of estrogen receptor expression activation or of estrogen production can prevent breast cancer in women at high risk. Similarly, oophorectomy also reduces cancer risk (21). BRCA1 and BRCA2 mutations are thought to reduce the capacity for DNA repair and therefore raise the risk of breast cancer, by increasing the probability of downstream genetic events associated with tumor progression. Because BRCA1 and BRCA2 are not in the main (i.e., estrogen dependent) pathway to tumorigenesis, they are not frequently mutated in sporadic tumors.
A comprehensive model for breast cancer. Applying a stem cell model to breast cancer helps explain much of the known data. The major risk factors are hormonal and can act to influence the number and activation of stem cells. Useful prevention strategies inhibit estrogen production or action. BRCA1 and BRCA2 germline mutations increase the likelihood of P53 inactivation (directly or through inhibition of DNA repair), but the BRCA genes are outside of the main pathway and thus are not found in most sporadic tumors.
For renewal tissues such as the colon or skin, the stem cells divide at a very slow rate. In the absence of perturbation of these tissues, cancer risk is very low. Activation of stem cells in renewal tissues can occur by inflammation, or tissue damage caused by radiation, mutagens, and irritants (Figure 2⇑). This chronic tissue damage leads to an accelerated division of stem cells, increasing the available number of target cells. In the colon, inflammatory diseases such as Crohn Disease and inflammatory bowel disease are risk factors for cancer, as are high fat/low fiber diets (22). Patients with the familial adenomatous polyposis coli (FAP) syndrome have large numbers of colon polyps. Because polyps are pre-malignant lesions, individuals that have polyps are at very high risk for colon cancer (23). Mutations in APC are responsible for FAP (24, 25) and these patients as well as mice with an equivalent genetic mutation exhibit increased cellular proliferation in the colon (26–28). The effect of APC mutations might be to increase the proliferation of colonic stem cells (3). Consistent with this model, APC is mutated in the vast majority of sporadic colon tumors (29). The later stage events in colon carcinogenesis are well known and involve mutations in P53, RAS, and other genes (29).
The major environmental risk factors can be classified by their role in either activating stem cells or contributing to the formation of a pre-malignant lesion (Table 2⇓). Many cancers in solid tissues are associated with agents causing inflammation or irritation of the cells in the tissue. Hepatitis B and C viruses are the principal risk factor for liver cancer, one of the most common cancers in the world (30, 31). There is no evidence that HBV or HCV contain genes that directly inactivate tumor suppressor genes; however, patients persistently infected with these viruses usually suffer from liver inflammation and extensive cirrhosis. The liver is known to be particularly adept at regeneration and repairing damaged tissue. Thus, persistent infection leads to chronic inflammation and tissue damage and continual stimulation of hepatic stem cells.
Genetic Aberrations Involved in Cancers Originating from Stem Cells
Asbestos is a metabolically inert compound remaining in the lungs of individuals exposed to large amounts of the fibers. Asbestos exposure causes asbestosis, a fibrotic disease of the lung. Asbestos exposure is also a risk factor for mesothelioma, a cancer of the mesothelial layer of the lung (32). Asbestos also works synergistically with tobacco in increasing the risk of other lung cancers (33). The chronic fibrosis and irritation caused by asbestos could be expected to continuously stimulate stem cells residing in the lung and lead to an increased risk of mutational events causing the formation of a pre-malignant lesion. Similar rationales can be invoked for the association of infection of the bacterium H. pylori and metal dust exposure with gastric cancer (34, 35) and the association of chronic pancreatitis and diabetes mellitus with pancreatic cancer (36).
Some agents associated with cancer may contribute both to stem cell activation as well as downstream mutations and tumor suppressor gene inactivation. Human papilloma viruses, particularly HPV16 and 18 are highly associated with cervical cancer (37). These viruses contain proteins (i.e., E6 and E7) that inhibit the p53 and RB1 tumor suppressor proteins (38, 39); however, most women with pathogenic HPV infection do not develop cervical cancer and so other factors are likely involved. HPV infection of cervical stem cells leads to the increased proliferation of these cells. Although HPV employs several strategies to minimize the immune response (40) and infected women do not have a visibly inflamed cervix, the immune system does seem to recognize HPV-infected cells because immunocompromised patients are at increased risk for cervical cancer (41). This chronic proliferation provides an increased pool of cells susceptible to viral integration and disease progression.
The associations of smoking and lung cancer and UV exposure and skin cancer are thought to principally arise from the mutagenic effects of these agents, and these tissues exhibit highly specific mutations (42). Tobacco smoke contains several mutagens and UV irradiation is clearly mutagenic. Indeed, both long-term tobacco use and long-term sun exposure are associated with chronic tissue inflammation and damage. These effects are likely to lead to an increased proliferation of tissue stem cells as the body tries to repair the damaged tissue. The constitutively dividing stem cells in these tissues would be targets for carcinogen-induced mutations, leading to tumorigenesis.
Research Implications
The cancer stem cell concept has profound implications for basic cancer research. While cancer biologists have long recognized the heterogeneity of tumor cells, they have assumed that cloned cells lines are homogeneous. We now know this to be untrue, and the analysis of tumor cell cultures involves the study of a large population of committed tumor cells and a small population of tumor stem cells. Thus, gene expression, microarray, proteomic, and many phenotypic assays may need to be reinterpreted. Most screens for cancer cytotoxic drugs have involved cell cultures treated over short time periods (43). A drug specifically inhibiting a tumor stem cell may show only modest activity in such an assay and not be chosen for follow up study. Clinical studies of stem cell inhibitors may need to be redesigned to capture long term benefits.
Through the use of specific cell surface markers tumor stem cells might be isolated from cell lines and characterized. Alternatively normal stem cells have been purified by taking advantage of the fact that stem cells express transport proteins that efflux certain dyes such as Hoechst and rhodamine. These so called side-population (SP) cells have been found in bone marrow and normal tissues, as well as in tumor cell lines (8, 9). The isolation of such tumor stem cells would likely lead to improved understanding of gene expression, cell surface proteins, and drug sensitivities of these cells. One long-recognized property of cancer cells is anchorage independence—as measured in vitro by the ability of tumor cells to proliferate into colonies in soft agar medium (2). Interestingly, the only normal cells with this property are stem cells, and only a fraction of cells in a tumor cell culture can form a colony in soft agar. This clonogenicity ranges from less that 1% to 20%–50% (Michael Alley, personal communication). A likely explanation of these data is that the only cells in a tumor cell line forming colonies are the tumor stem cells, the rest of the cells in the tumor being committed to differentiation or senescence. In fact, the fraction of (SP) cells in tumor cell lines correlates with their clonogenicty, in the few lines that have been examined [data not shown but reported in (9)]. An exciting implication of this result is that by screening for drugs suppressing colony formation, we can identify drugs that inhibit the proliferation of tumor stem cells. Such an assay would be more cumbersome and have a lower throughput than screens on adherent cells but could prove to be more informative.
Stem cells, especially germ cells, possess the ability to migrate throughout the developing body. This is particularly true of cells derived from the neural crest that give rise to melanoma, neuroblastoma, and small cell lung cancers. Most of the mortality of cancer arises from the metastasis of cells from the original tumor site, and their migration to other organs. Could the metastatic ability of some tumor cells relate to an innate property of stem cells to migrate? If so, then further understanding of germ cell migration could lead to new insights into metastasis.
Treatment Implications
In the light of a stem cell model, tumors that regress following radiation and chemotherapy protocols are not the result of a rare cell from within the tumor but are the regrowth of the cancer stem cells in the original tumor. However, tumor stem cells could acquire additional genetic changes to render them even more drug resistant, radiation resistant, or aneuploid. Because cures are achieved for many types of cancer, cancer stem cells must be eliminated by some therapeutic strategies. The committed cells in a tumor may very well play a role in supporting or stimulating the stem cells, and regression of the committed cells in a tumor could lead to elimination of the stem cells as well. Immune surveillance is clearly important in many cancers (44), and decreasing the mass of the tumor may allow the immune system to efficiently recognize the remaining cells. Clearly, targeted therapies that directly suppress or kill the tumor stem cells may have increased efficacy. For example, angiogenesis is likely to be critical to provide blood supply to the tumor stem cells, so strategies to fight the development or inhibit the tumors blood vessels are likely to be effective (45).
Therapies that target cancer stem cells, however, may require new approaches to evaluating the success of a cancer agent. If a drug only inhibits the proliferation of the cancer stem cells (rather than killing them outright) the drug will affect the growth of only a minor population of cells and there may be a minimal decrease in the growth of the tumor in the short term. Pharmacodynamic assays to demonstrate the drug is inhibiting the target protein will be important and the use of endpoints such as disease free survival may be useful. Cancer stem cell–targeted therapies will no doubt be combined with standard therapies, to attempt the complete elimination of the tumor. As with all cancer therapies it will be critical to establish a dose and schedule where the tumor is suppressed or eliminated without undue toxicity of normal stem cells. Recent data on mouse leukemia models suggest that such therapies may be established (46).
One of the innate resistance mechanisms of stem cells is the expression of one or more ATP-binding cassette (ABC) transporters. These pumps play a role in protecting stem cells from xenobiotic toxins (47). ABCG2 and ABCB1/MDR1 genes are expressed in the vast majority of stem cells and in most tumor stem cells (11–13). These transporters can efflux fluorescent dyes such as rhodamine and Hoecht 33342, and this property allows the stem cells to be separated from non-stem cells on a cell sorter (48). The combined use of chemotherapy drugs and ABC transporter inhibitors could be used to specifically target cancer stem cells (49, 50). In fact, there are highly specific inhibitors of ABCB1 in clinical use and ABCG2 inhibitors in development (51). These therapies would be predicted to have toxic effects on the patient’s normal stem cells, and both ABCG2 and ABCB1 play a role in the blood-brain barrier, which suggests that this approach would have to be carefully titrated to avoid excessive toxicity.
Directly targeting the growth of stem cells could be a fruitful avenue. Because of ground-breaking work in Drosophila and other developmental systems, we know a great deal about the growth regulatory pathways operative in embryonic cells (52). One such pathway, involving the Hedgehog (HH) and WNT signaling molecules, contains a large number of genes that can act as tumor suppressor genes or oncogenes in mammalian cells (5). For example, Patched (PTCH) codes for the receptor that binds HH molecules and is mutated in patients with nevoid basal cell carcinoma syndrome (53, 54). PTCH is also mutated in nearly all sporadic basal cell carcinomas and in some medulloblastomas (55). The mammalian HH genes (sonic hedgehog (SHH), Indian hedgehog (IHH), and desert hedgehog (DHH)) are overexpressed in a wide variety of cancers, including small-cell lung, pancreas, gastric, breast, and prostate (56–59). HH family overexpression and PTCH mutation both have the effect of constitutive action of smoothened (SMO) a G protein–coupled receptor that is a key signaling component of the pathway. Constitutive HH family expression could lead to stem cell activation and appears to be a common feature of many cancers.
Cyclopamine is a compound originally discovered in the Corn Lily (Veratrum californicum), a plant teratogenic to sheep (60). Cyclopamine specifically inhibits the SMO protein and can suppress the growth of cells and tumors that contain activated HH (61). Human prostate tumors grown as xenografts in mice were completely eliminated following twenty-one days of treatment with cyclopamine (59), and UV-induced basal cell carcinomas were suppressed in mice given low levels of cyclopamine in their drinking water (62). Both cyclopamine and SMO inhibitors are in development as anti-cancer agents.
The Notch protein is another protein important to the growth and differentiation of stem cells. Notch is processed by the enzyme γ-secretase, the same enzyme that processes the APP protein important in Alzheimer Disease. Several companies have developed γ-secretase inhibitors and these drugs may have applications to cancer therapy. Rapamycin is a bacterially derived molecule that inhibits the target of rapamycin (TOR) family of kinases (63). Rapamycin has immunosuppressive properties and is also used to treat certain leukemias (64). Yilmaz et al. have shown that rapamycin can selectively inhibit leukemia initiating cells in leukemias generated in mice harboring a conditional deletion of PTEN (phosphatase and tensin homolog deleted on chromosome 10), a phosphati-dylinositol phosphate (PIP) phosphatase often mutated in human tumors (46). Rapamycin depletes the leukemia-initiating cells while restoring the normal hematopoieitc stem cells, suggesting therapies can be devised specific for cancer stem cells. Additional strategies to directly target the growth of tumor stem cells can now be developed and may prove superior in effectiveness.
Nearly all cancer mortality derives from metastatic leasions, not the primary tumor. Therefore it is of great importance to understand the nature of metastases and how to inhibit or eliminate them. It follows from the cancer stem cell model that metastatic lesions must initiate from a cancer stem cell. In fact many stem cells, such as those in the neural crest, migrate through the developing body. Thus the migratory behavior that we recognize as metastasis may be a “normal” function of the cancer stem cell. Therefore drugs that inhibit the growth of cancer stem cells may have the effect of suppressing metastasis. Although the ultimate goal of cancer therapy is the complete elimination of the tumor, turning cancer from an acute disease to a chromic disease may be a more achievable near-term prospect.
Conclusions
The concept of cancer as a stem cell disease has the potential to dramatically change our view of the problem. By separating the disease into a stem cell activation phase and a tumor progression phase, historical cancer studies can be reinterpreted with new understanding. Research efforts to understand the growth of tumor stem cells as well as identify tumor stem cell antigens could lead to new targeted approaches. Cancer diagnostics, prevention, and therapeutics are likely to be greatly aided by this new insight. Normal stem cells are being intensively studied to develop approaches for replacing damaged cells and tissues in the body. The insight from this work is likely to aid the understanding of cancer stem cells.
- © American Society for Pharmacology and Experimental Theraputics 2006
References
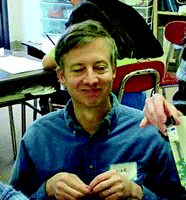
Michael Dean, PhD, received his doctorate degree from the Biochemistry Department at the Boston University School of Medicine. His advisor was Dr. Gail Sonenshein and he studied transcription and regulation of the MYC oncogene. He performed his postdoctoral studies at the NCI-Frederick with Dr. George Vande Woude where he studied the MET oncogene. They discovered linkage of MET to the locus for cystic fibrosis. After joining the Laboratory of Genomic Diversity (LGD), Dr. Dean’s group participated in the molecular cloning of the cystic fibrosis gene, identification of variants in CCR5 and CCR2 involved in HIV-1 infection and AIDS progression, identification of the VHL and PTCH genes in inherited cancers, and the involvement of several ATP-binding cassette (ABC) transporters in human disease and drug resistance. He is shown here teaching as part of the Elementary Outreach Program. This is an effort Michael created bringing NCI and contract employees into Frederick County elementary schools. Over 7,000 students have received hands-on science lessons in the six years the program has been operating. In his spare time Michael enjoys kayaking, skiing and playing softball. He is Chief of the Human Genetics Section in the LGD. E-mail: dean{at}ncifcrf.gov; fax: 301-846-1909.